Soil CArbon and Carbon Credits
YLAD Living Soils Seminars: Eurongilly - 14 February, Young - 15 February 2006
Aggregate or aggravate? Creating soil carbon
Christine Jones
Organic carbon is the basic building block for all life on - and in - the earth. We cannot live without it. Neither can our soils.
Carbon (C) provides the structural basis for thousands of different compounds. It is so common, we take it for granted. We often take hydrogen (H) and oxygen (O) for granted too - but where would we be without H2O – our precious, life sustaining water? The significance of soil water is becoming more apparent as we lose soil carbon. Low soil moisture and low levels of soil organic carbon go hand in hand.
Organic carbon begins and ends its journey as a gas, carbon dioxide (CO2). Atmospheric carbon is an extremely valuable resource. When sequestered in topsoil as organic carbon, it brings with it a wealth of environmental, productivity and quality of life benefits. An understanding of the ‘carbon cycle’ and the role of carbon in soils, is essential to our understanding of life on earth.
In the miracle of photosynthesis, which takes place in the chloroplasts of green leaves, carbon dioxide (CO2) from the air and water (H2O) from the soil, combine to capture sunlight energy and store it in the form of a simple sugar - glucose (C6H12O6). Through a myriad of chemical reactions, this glucose forms the basis of a great diversity of carbon compounds, including carbohydrates, proteins, organic acids, humic substances, waxes and oils – and our ‘fossil fuels’ coal, oil and gas. We have a great deal to thank green leaves for!!
Dinosaurs
Liquorice allsorts
The clothes we wear
Me and you
All are carbon atoms in various patterns
Amazing what carbon can do!!!
The reason there are so many carbon compounds is that carbon atoms can link together to form long chains, branched chains and rings, to which other elements, such as hydrogen and oxygen, can join. The study of carbon compounds is called organic chemistry.
Some carbon compounds are complex, while others are very simple. Gases like methane and ethane, and liquids like petrol, belong to a family called hydrocarbons, that is, carbon combined with hydrogen. The gas, carbon dioxide (CO2), is simply carbon and oxygen. When carbon joins with both hydrogen and oxygen, it forms fats and oils, as well as carbohydrates such as the sugars and starches found in a great variety of breads, cereals, vegetables and fruit. Proteins are formed from carbon, hydrogen, oxygen and nitrogen and often include other elements such as sulphur and phosphorus.
Representatives of all groups of carbon compounds are found in soils. These compounds are essential to the creation of ‘topsoil’ from the structureless, lifeless ‘mineral soil’ produced by the weathering of rocks.
Sadly, around 50 – 80% of the organic carbon that was once in the topsoil has been lost to the atmosphere over the last 150 years or so, due to our failure to take care of the earth as a living thing.
By inference, degraded soils have the potential to store up to five (5) times more organic carbon in their surface layers than they currently hold, provided we change the way we manage the land.
A net gain of organic carbon in soils is win-win for plants, animals and people. A net gain of carbon in the atmosphere is lose-lose. Our role, as managers of the carbon cycle, is to ensure that as much carbon as possible is returned to soils and as little as possible goes into the air.
When we consider how much carbon has been lost from soil, and the implications for our current ‘problems’, such as global warming, global dimming, soil erosion, sedimentation in rivers and oceans, soil acidity, soil salinity …
….. we realise IT”S TIME
….. it’s time we put the carbon back!!!
As described earlier, the ‘way in’ for soil carbon is the process of photosynthesis in green leaves. The cheapest, most efficient and most beneficial form of organic carbon for soil life is exudation from the actively growing roots of plants in the Poaceae family, which includes pasture grasses and cereals. The breakdown of their fibrous roots is also an important source of carbon in soils. Organic carbon additions are governed by the volume of plant roots per unit of soil and their rate of growth. The more active plant roots there are, the more carbon is added. It’s as simple as that.
Many of the benefits of Pasture Cropping and Advance Sowing, for example, can be attributed to having perennial pasture grasses and cereals together, side by side in space and time. The ongoing carbon additions from the perennial grass component evolve into highly stable forms of soil carbon, while the short-term, high sugar forms of carbon exuded by the roots of the cereal crop stimulate microbial activity.
In this positive feedback loop, CO2 respired by plant roots and aerobic soil microbes, slowly moves upwards through the topsoil and increases the partial pressure of CO2 beneath the crop/pasture canopy, enhancing photosynthetic potential. As money makes money - so carbon makes carbon – but only when the groundcover management is right.
Under conventional cropping or annual pastures, the stimulatory exudates from short-lived plants are negated by bare earth, cultivation and harsh chemicals. Over time, organic carbon levels in soils subjected to these kinds of agricultural practices fall to levels where the soil is essentially ‘dead’.
We once farmed Fields of Eden, we grew our food with style
It’s time to stop, and look, and listen for a while
Earth lying naked and barren
Crying for help without words
Calling so softly for carbon
There is no time now to bargain
Under regenerative regimes, soil carbon and soil life are restored, conferring multiple ecological and production benefits in terms of nutrient cycling, soil water storage, soil structural integrity and disease suppression. Benefits extend well beyond the paddock gate. Improved soil and water quality are the ‘key’ to catchment health, while carbon sequestration in soil is the most potent mechanism available for reducing greenhouse gases and mitigating climate change.
In addition to root exudation, soil carbon levels can also be increased using principles employed in the rapidly expanding arena of ecological agriculture, which includes biodynamic, organic and biological farming. There are many and varied practices including the use of cover crops, green manures, mulches, worm juice, fish and seaweed products, animal manures, recycled greenwaste, biosolids, composts, compost teas, liquid injection, humic substances, microbial stimulants and innumerable combinations thereof.
Adding organic carbon to soil is one thing. Keeping it there is another. Topsoil is always in a state of dynamic equilibrium with the atmosphere. Carbon additions therefore need to be combined with land management practices that foster the conversion of relatively transient forms of organic carbon to more stable complexes within the soil.
Carbon sources and carbon sinks
In bare paddocks, or cropped or grazed paddocks dominated by annual plants, more carbon will move to the atmosphere than is sequestered. That is, the soil is said to be a SOURCE of atmospheric carbon. This adds substantially to the accumulation of the greenhouse gases responsible for global warming and climate change.
Anything that causes bare ground results in the loss of organic carbon. Even conventional afforestation and reforestation practices result in net losses in soil carbon for several decades, due to the lack of groundcover under the trees. If bare earth is produced by chemical or mechanical means, we add insult to injury by burning fossil carbon and adding that to the atmosphere as well.
In cropped or grazed paddocks managed regeneratively, actively forming topsoils act as carbon SINKS. That is, more carbon is sequestered than is lost, reducing the level of carbon dioxide in the atmosphere.
Stable soil carbon
Organic carbon moves between various ‘pools’ in the soil, some of which are short lived while others may persist for thousands of years. Glomalin and humic substances are two of the relatively stable forms of soil carbon. Their creation and destruction are strongly influenced by land management.
Glomalin
The relatively recent discovery of glomalin has caused a complete re-examination of what makes up soil organic matter and how we measure it. Glomalin is a glycoprotein (contains both protein and carbohydrate) produced by arbuscular mycorrhizal fungi living on plant roots. Most current soil chemical extraction techniques greatly underestimate the amount of glomalin, which can persist for several decades and may account for one third of the organic carbon stored in agricultural soils.
Prior to the discovery of glomalin, humic acid was thought to be the main contributor to soil carbon. Dr Sara Wright (USDA – ARS) has shown that glomalin contributes about seven times more carbon to total soil organic carbon than do humic acids. This discovery has attracted a great deal of interest from a carbon storage and carbon trading perspective, in addition to the multiple benefits for soil and catchment health.
Glomalin forms on the outer surface of the hyphae of mycorrhizal fungi, which colonise the roots of certain plants. In everyday terms, the more of these roots there are and the more active they are, the more glomalin can be produced. The glomalin molecule also contains quite high levels of iron which are thought to help protect plants from soil pathogens.
Most members of the grass family are excellent hosts for mycorrhizal fungi, with up to 100 metres of microscopic fungi forming per gram of soil under healthy grassland. Enhanced glomalin formation may partly explain the success of Pasture Cropping and Advance Sowing.
Inhibitory factors for mycorrhizal fungi and glomalin production include bare soil, intensive tillage, the application of phosphorus fertiliser and the presence of plants from the Brassica family, such as canola, turnips, cabbage and broccoli, which do not form mycorrhizal associations.
Humus
There is nothing recent about the discovery of humus, which dates back to Roman times. Despite being the best known of the stable organic fractions in soils, humus has never been clearly defined or understood. Even today, some aspects of the formation, composition, structure and function of the heterogeneous group of high molecular weight humic substances known collectively as ‘humus,’ remains somewhat of a mystery.
One reason is that humus is an integral component of the soil matrix and cannot be successfully isolated for scientific research. Synthetic humus or extracted humus does NOT have the same properties as humus found naturally within the soil.
There are many theories on the formation of humus, most of which suggest a microbial resynthesis and polymerisation of carbon compounds derived from organic matter. If soil conditions are conducive to biological activity however, levels of humus can increase to a greater extent than would be possible from the weight of organic materials grown or applied. This suggests that microbial stimulation per se may be the factor of greatest importance, with organic matter simply fuelling the process.
In healthy soils, stable humic substances can persist for over one thousand years. Bare earth and practices that destroy soil structure, such as intensive tillage or the application of anhydrous ammonia, result in the loss of humus.
Humic substances have significance above and beyond the relatively long-term sequestration of atmospheric carbon. They are extremely important in terms of pH buffering, inactivation of pesticides and other pollutants, improved plant nutrition and increased water-holding capacity of soil. Humic substances can also effectively ameliorate the symptoms of dryland salinity by chelating salts and stimulating biological activity.
Loss of humus therefore has a highly significant effect on the health and productivity of soil.
Carbon credits
The capacity for appropriately managed soils to sequester atmospheric carbon is enormous. The world’s soils hold around twice as much carbon as the atmosphere and almost three times as much carbon as the vegetation. Soil represents the largest carbon sink over which we have control. Improvements in soil carbon levels could be made in all rural areas, whereas the regions suited to carbon sequestration in plantation timber are limited.
If financial incentives in the form of ‘carbon credits’ amounting to several thousand dollars per hectare became the primary focus of primary production, farm enterprises such as meat, wool or grain could become of secondary importance as an income source. This would reduce the potential for destructive farm practices and provide a large incentive for ‘greener’ forms of agriculture.
Australia has the highest per capita rate of greenhouse gas emissions in the world. Appropriately managed farmlands could effectively ‘mop up’ most of the excess carbon being emitted to the atmosphere, converting a potential hazard into an extremely productive opportunity.
Calculating soil carbon
Soil carbon content is usually expressed as either a concentration (%) or a stock (t/ha). Unless the depth of measurement and soil bulk density parameters are known, it is not possible to accurately convert from one unit of measurement to the other.
For the sake of illustration however, some simple assumptions can be made. Changes in the stock of soil carbon (t/ha) for each 1% change in measured organic carbon (OC) status for a range of soil bulk densities and measurement depths are shown in Table 1. Numbers in brackets represent tCO2 equivalent. An explanation of these terms follows.
Soil bulk density (g/cm3) is the dry weight (g) of one cubic centimetre (cm3) of soil. The higher the bulk density the more compact the soil. Generally, soils of low bulk density are well structured and have ‘more space than stuff’. The lower the bulk density the more room for air and water and the better the conditions for soil life and nutrient cycling. Bulk density usually increases with soil depth. To simplify the table it was assumed that soil bulk density did not change with depth
CO2 equivalent. Every tonne of carbon lost from soil adds 3.67 tonnes of carbon dioxide (CO2) gas to the atmosphere. Conversely, every 1 t/ha increase in soil organic carbon represents 3.67 tonnes of CO2 sequestered from the atmosphere and removed from the greenhouse gas equation.
For example, from TABLE 1 we can see that a 1% increase in organic carbon in the top 20 cm of soil with a bulk density of 1.2 g/cm3 represents a 24 t/ha increase in soil OC which equates to 88 t/ha of CO2 sequestered.
TABLE 1. Changes in the stock of soil carbon (tC/ha) for each 1% change in measured organic carbon (OC) status for a range of soil bulk densities and measurement depths. Numbers in brackets represent tCO2 equivalent.
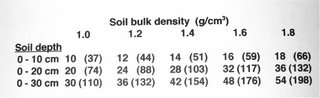
Value of soil carbon. Sequestered carbon is a tradeable commodity. It has different values in different markets and the price is subject to market fluctuation. If the CO2 equivalent in the above example was worth $15/t, the value of sequestered soil carbon in ‘carbon credits’ would be $1,056/ha. If the soil carbon concentration was increased by 1% to a depth of 30cm rather than to 20 cm, this would represent 132 t/ha sequestered CO2 at a value of $1,980/ha.
If organic carbon concentrations were increased by 2% to a depth of 30 cm in the same example, this would represent $3,960/ha, that is, almost $400,000 in ‘carbon credits’ per 100 ha of regenerated land. These levels of increase in soil carbon are achievable, and have already been achieved, by landholders practicing regenerative cropping and grazing practices.
Even if organic carbon levels were only increased by 0.5% in the top 10 cm of soil this would represent 22 t/ha sequestered CO2 valued at $33,000 per 100 ha regenerated land (assuming a soil bulk density of 1.2 g/cm3 and a price of $15/t CO2 equivalent).
Carbon credits for sequestered carbon are not an annual payment. In order to receive further credits, the level of soil carbon would need to be further increased. It is also important that the OC level for which payment was received is maintained.
This is not difficult with regenerative regimes in which new topsoil is being formed. Biological activity is concentrated in the top 10cm of most agricultural soils, but regenerative practices rapidly expand this activity zone to 30 cm and deeper. Many benefits in addition to potential carbon credits accrue to increased root biomass and increased levels of biological activity in soil.
The majority of Australian soils have lost enormous quantities of organic carbon and this process needs to be reversed. What has gone up must come down. Soils, plants, animals and people will benefit when we take ‘recycle and re-use’ to the next logical step and recycle the excess carbon currently in the atmosphere.
Carbon and nitrogen
Nitrogen moves between the atmosphere and the topsoil in similar ways to carbon. The main difference is that the ‘way in’ for atmospheric carbon is via green plants whereas the ‘way in’ for atmospheric nitrogen is soil microbes. Soils acting as net sinks for carbon are usually also acting as net sinks for nitrogen. The flip side is that soils losing carbon are usually losing nitrogen too. In poorly aerated soils, some of this loss is in the form of nitrous oxide (N2O), a greenhouse gas up to 300 times more potent than carbon dioxide, while other losses include easily leached nitrate (NO3-) which often takes calcium, magnesium and potassium with it, leaving the soil more acidic (lower pH).
Rewarding landholders for farming in ways that build new topsoil and raise levels of soil carbon and nitrogen would have a significant impact on the vitality and productivity of Australia’s rural industries, reduce the incidence of dryland salinity and soil acidity – and reduce levels of greenhouse gases.
As a bonus, regenerative farming practices result in the production of food much higher in vitamin and mineral content and lower in herbicide and pesticide residues than conventionally produced foods.
A new era
If landholders were rewarded for regenerative practices that aggregate rather than aggravate soil structure, it would move us a long way towards solving the greenhouse gas ‘problem’, without the need to measure soil carbon levels at all. Any farming practice that improves soil structure is building soil carbon. When soils become light, soft and springy, easier to dig or till and less prone to erosion, waterlogging or dryland salinity - then organic carbon levels are increasing. If soils are becoming more compact, eroded or saline – organic carbon levels are falling.
Water, energy, life, nutrients and profit will increase on-farm as soil organic carbon levels rise. The alternative is evaporation of water, energy, life, nutrients and profit if carbon is mismanaged and goes into the air.
It’s about turning carbon loss into carbon gain.
Getting started in lifeless, compacted soils where the soil engine has shut down is the hard part. The longer we delay, the more difficult it will be to re-sequester soil carbon and balance the greenhouse equation
Our soils were rich in carbon, life’s treasures flourished there
Then soil health we neglected: earth’s carbon moved to air
Everywhere climates are changing
The future’s becoming unclear
Warming us slowly with carbon
There is no time now to bargain
………………………………………………………………………….
Further information:
‘Managing the Carbon Cycle’ Forums will be held in Horsham, VIC, 26-27 July 2006; Katanning, WA, 2-3 August 2006 and Kingaroy, QLD, 25-26 October 2006. See www.amazingcarbon.com or contact Christine@amazingcarbon.com
Aggregate or aggravate? Creating soil carbon
Christine Jones
Organic carbon is the basic building block for all life on - and in - the earth. We cannot live without it. Neither can our soils.
Carbon (C) provides the structural basis for thousands of different compounds. It is so common, we take it for granted. We often take hydrogen (H) and oxygen (O) for granted too - but where would we be without H2O – our precious, life sustaining water? The significance of soil water is becoming more apparent as we lose soil carbon. Low soil moisture and low levels of soil organic carbon go hand in hand.
Organic carbon begins and ends its journey as a gas, carbon dioxide (CO2). Atmospheric carbon is an extremely valuable resource. When sequestered in topsoil as organic carbon, it brings with it a wealth of environmental, productivity and quality of life benefits. An understanding of the ‘carbon cycle’ and the role of carbon in soils, is essential to our understanding of life on earth.
In the miracle of photosynthesis, which takes place in the chloroplasts of green leaves, carbon dioxide (CO2) from the air and water (H2O) from the soil, combine to capture sunlight energy and store it in the form of a simple sugar - glucose (C6H12O6). Through a myriad of chemical reactions, this glucose forms the basis of a great diversity of carbon compounds, including carbohydrates, proteins, organic acids, humic substances, waxes and oils – and our ‘fossil fuels’ coal, oil and gas. We have a great deal to thank green leaves for!!
Dinosaurs
Liquorice allsorts
The clothes we wear
Me and you
All are carbon atoms in various patterns
Amazing what carbon can do!!!
The reason there are so many carbon compounds is that carbon atoms can link together to form long chains, branched chains and rings, to which other elements, such as hydrogen and oxygen, can join. The study of carbon compounds is called organic chemistry.
Some carbon compounds are complex, while others are very simple. Gases like methane and ethane, and liquids like petrol, belong to a family called hydrocarbons, that is, carbon combined with hydrogen. The gas, carbon dioxide (CO2), is simply carbon and oxygen. When carbon joins with both hydrogen and oxygen, it forms fats and oils, as well as carbohydrates such as the sugars and starches found in a great variety of breads, cereals, vegetables and fruit. Proteins are formed from carbon, hydrogen, oxygen and nitrogen and often include other elements such as sulphur and phosphorus.
Representatives of all groups of carbon compounds are found in soils. These compounds are essential to the creation of ‘topsoil’ from the structureless, lifeless ‘mineral soil’ produced by the weathering of rocks.
Sadly, around 50 – 80% of the organic carbon that was once in the topsoil has been lost to the atmosphere over the last 150 years or so, due to our failure to take care of the earth as a living thing.
By inference, degraded soils have the potential to store up to five (5) times more organic carbon in their surface layers than they currently hold, provided we change the way we manage the land.
A net gain of organic carbon in soils is win-win for plants, animals and people. A net gain of carbon in the atmosphere is lose-lose. Our role, as managers of the carbon cycle, is to ensure that as much carbon as possible is returned to soils and as little as possible goes into the air.
When we consider how much carbon has been lost from soil, and the implications for our current ‘problems’, such as global warming, global dimming, soil erosion, sedimentation in rivers and oceans, soil acidity, soil salinity …
….. we realise IT”S TIME
….. it’s time we put the carbon back!!!
As described earlier, the ‘way in’ for soil carbon is the process of photosynthesis in green leaves. The cheapest, most efficient and most beneficial form of organic carbon for soil life is exudation from the actively growing roots of plants in the Poaceae family, which includes pasture grasses and cereals. The breakdown of their fibrous roots is also an important source of carbon in soils. Organic carbon additions are governed by the volume of plant roots per unit of soil and their rate of growth. The more active plant roots there are, the more carbon is added. It’s as simple as that.
Many of the benefits of Pasture Cropping and Advance Sowing, for example, can be attributed to having perennial pasture grasses and cereals together, side by side in space and time. The ongoing carbon additions from the perennial grass component evolve into highly stable forms of soil carbon, while the short-term, high sugar forms of carbon exuded by the roots of the cereal crop stimulate microbial activity.
In this positive feedback loop, CO2 respired by plant roots and aerobic soil microbes, slowly moves upwards through the topsoil and increases the partial pressure of CO2 beneath the crop/pasture canopy, enhancing photosynthetic potential. As money makes money - so carbon makes carbon – but only when the groundcover management is right.
Under conventional cropping or annual pastures, the stimulatory exudates from short-lived plants are negated by bare earth, cultivation and harsh chemicals. Over time, organic carbon levels in soils subjected to these kinds of agricultural practices fall to levels where the soil is essentially ‘dead’.
We once farmed Fields of Eden, we grew our food with style
It’s time to stop, and look, and listen for a while
Earth lying naked and barren
Crying for help without words
Calling so softly for carbon
There is no time now to bargain
Under regenerative regimes, soil carbon and soil life are restored, conferring multiple ecological and production benefits in terms of nutrient cycling, soil water storage, soil structural integrity and disease suppression. Benefits extend well beyond the paddock gate. Improved soil and water quality are the ‘key’ to catchment health, while carbon sequestration in soil is the most potent mechanism available for reducing greenhouse gases and mitigating climate change.
In addition to root exudation, soil carbon levels can also be increased using principles employed in the rapidly expanding arena of ecological agriculture, which includes biodynamic, organic and biological farming. There are many and varied practices including the use of cover crops, green manures, mulches, worm juice, fish and seaweed products, animal manures, recycled greenwaste, biosolids, composts, compost teas, liquid injection, humic substances, microbial stimulants and innumerable combinations thereof.
Adding organic carbon to soil is one thing. Keeping it there is another. Topsoil is always in a state of dynamic equilibrium with the atmosphere. Carbon additions therefore need to be combined with land management practices that foster the conversion of relatively transient forms of organic carbon to more stable complexes within the soil.
Carbon sources and carbon sinks
In bare paddocks, or cropped or grazed paddocks dominated by annual plants, more carbon will move to the atmosphere than is sequestered. That is, the soil is said to be a SOURCE of atmospheric carbon. This adds substantially to the accumulation of the greenhouse gases responsible for global warming and climate change.
Anything that causes bare ground results in the loss of organic carbon. Even conventional afforestation and reforestation practices result in net losses in soil carbon for several decades, due to the lack of groundcover under the trees. If bare earth is produced by chemical or mechanical means, we add insult to injury by burning fossil carbon and adding that to the atmosphere as well.
In cropped or grazed paddocks managed regeneratively, actively forming topsoils act as carbon SINKS. That is, more carbon is sequestered than is lost, reducing the level of carbon dioxide in the atmosphere.
Stable soil carbon
Organic carbon moves between various ‘pools’ in the soil, some of which are short lived while others may persist for thousands of years. Glomalin and humic substances are two of the relatively stable forms of soil carbon. Their creation and destruction are strongly influenced by land management.
Glomalin
The relatively recent discovery of glomalin has caused a complete re-examination of what makes up soil organic matter and how we measure it. Glomalin is a glycoprotein (contains both protein and carbohydrate) produced by arbuscular mycorrhizal fungi living on plant roots. Most current soil chemical extraction techniques greatly underestimate the amount of glomalin, which can persist for several decades and may account for one third of the organic carbon stored in agricultural soils.
Prior to the discovery of glomalin, humic acid was thought to be the main contributor to soil carbon. Dr Sara Wright (USDA – ARS) has shown that glomalin contributes about seven times more carbon to total soil organic carbon than do humic acids. This discovery has attracted a great deal of interest from a carbon storage and carbon trading perspective, in addition to the multiple benefits for soil and catchment health.
Glomalin forms on the outer surface of the hyphae of mycorrhizal fungi, which colonise the roots of certain plants. In everyday terms, the more of these roots there are and the more active they are, the more glomalin can be produced. The glomalin molecule also contains quite high levels of iron which are thought to help protect plants from soil pathogens.
Most members of the grass family are excellent hosts for mycorrhizal fungi, with up to 100 metres of microscopic fungi forming per gram of soil under healthy grassland. Enhanced glomalin formation may partly explain the success of Pasture Cropping and Advance Sowing.
Inhibitory factors for mycorrhizal fungi and glomalin production include bare soil, intensive tillage, the application of phosphorus fertiliser and the presence of plants from the Brassica family, such as canola, turnips, cabbage and broccoli, which do not form mycorrhizal associations.
Humus
There is nothing recent about the discovery of humus, which dates back to Roman times. Despite being the best known of the stable organic fractions in soils, humus has never been clearly defined or understood. Even today, some aspects of the formation, composition, structure and function of the heterogeneous group of high molecular weight humic substances known collectively as ‘humus,’ remains somewhat of a mystery.
One reason is that humus is an integral component of the soil matrix and cannot be successfully isolated for scientific research. Synthetic humus or extracted humus does NOT have the same properties as humus found naturally within the soil.
There are many theories on the formation of humus, most of which suggest a microbial resynthesis and polymerisation of carbon compounds derived from organic matter. If soil conditions are conducive to biological activity however, levels of humus can increase to a greater extent than would be possible from the weight of organic materials grown or applied. This suggests that microbial stimulation per se may be the factor of greatest importance, with organic matter simply fuelling the process.
In healthy soils, stable humic substances can persist for over one thousand years. Bare earth and practices that destroy soil structure, such as intensive tillage or the application of anhydrous ammonia, result in the loss of humus.
Humic substances have significance above and beyond the relatively long-term sequestration of atmospheric carbon. They are extremely important in terms of pH buffering, inactivation of pesticides and other pollutants, improved plant nutrition and increased water-holding capacity of soil. Humic substances can also effectively ameliorate the symptoms of dryland salinity by chelating salts and stimulating biological activity.
Loss of humus therefore has a highly significant effect on the health and productivity of soil.
Carbon credits
The capacity for appropriately managed soils to sequester atmospheric carbon is enormous. The world’s soils hold around twice as much carbon as the atmosphere and almost three times as much carbon as the vegetation. Soil represents the largest carbon sink over which we have control. Improvements in soil carbon levels could be made in all rural areas, whereas the regions suited to carbon sequestration in plantation timber are limited.
If financial incentives in the form of ‘carbon credits’ amounting to several thousand dollars per hectare became the primary focus of primary production, farm enterprises such as meat, wool or grain could become of secondary importance as an income source. This would reduce the potential for destructive farm practices and provide a large incentive for ‘greener’ forms of agriculture.
Australia has the highest per capita rate of greenhouse gas emissions in the world. Appropriately managed farmlands could effectively ‘mop up’ most of the excess carbon being emitted to the atmosphere, converting a potential hazard into an extremely productive opportunity.
Calculating soil carbon
Soil carbon content is usually expressed as either a concentration (%) or a stock (t/ha). Unless the depth of measurement and soil bulk density parameters are known, it is not possible to accurately convert from one unit of measurement to the other.
For the sake of illustration however, some simple assumptions can be made. Changes in the stock of soil carbon (t/ha) for each 1% change in measured organic carbon (OC) status for a range of soil bulk densities and measurement depths are shown in Table 1. Numbers in brackets represent tCO2 equivalent. An explanation of these terms follows.
Soil bulk density (g/cm3) is the dry weight (g) of one cubic centimetre (cm3) of soil. The higher the bulk density the more compact the soil. Generally, soils of low bulk density are well structured and have ‘more space than stuff’. The lower the bulk density the more room for air and water and the better the conditions for soil life and nutrient cycling. Bulk density usually increases with soil depth. To simplify the table it was assumed that soil bulk density did not change with depth
CO2 equivalent. Every tonne of carbon lost from soil adds 3.67 tonnes of carbon dioxide (CO2) gas to the atmosphere. Conversely, every 1 t/ha increase in soil organic carbon represents 3.67 tonnes of CO2 sequestered from the atmosphere and removed from the greenhouse gas equation.
For example, from TABLE 1 we can see that a 1% increase in organic carbon in the top 20 cm of soil with a bulk density of 1.2 g/cm3 represents a 24 t/ha increase in soil OC which equates to 88 t/ha of CO2 sequestered.
TABLE 1. Changes in the stock of soil carbon (tC/ha) for each 1% change in measured organic carbon (OC) status for a range of soil bulk densities and measurement depths. Numbers in brackets represent tCO2 equivalent.
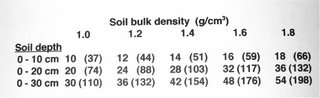
Value of soil carbon. Sequestered carbon is a tradeable commodity. It has different values in different markets and the price is subject to market fluctuation. If the CO2 equivalent in the above example was worth $15/t, the value of sequestered soil carbon in ‘carbon credits’ would be $1,056/ha. If the soil carbon concentration was increased by 1% to a depth of 30cm rather than to 20 cm, this would represent 132 t/ha sequestered CO2 at a value of $1,980/ha.
If organic carbon concentrations were increased by 2% to a depth of 30 cm in the same example, this would represent $3,960/ha, that is, almost $400,000 in ‘carbon credits’ per 100 ha of regenerated land. These levels of increase in soil carbon are achievable, and have already been achieved, by landholders practicing regenerative cropping and grazing practices.
Even if organic carbon levels were only increased by 0.5% in the top 10 cm of soil this would represent 22 t/ha sequestered CO2 valued at $33,000 per 100 ha regenerated land (assuming a soil bulk density of 1.2 g/cm3 and a price of $15/t CO2 equivalent).
Carbon credits for sequestered carbon are not an annual payment. In order to receive further credits, the level of soil carbon would need to be further increased. It is also important that the OC level for which payment was received is maintained.
This is not difficult with regenerative regimes in which new topsoil is being formed. Biological activity is concentrated in the top 10cm of most agricultural soils, but regenerative practices rapidly expand this activity zone to 30 cm and deeper. Many benefits in addition to potential carbon credits accrue to increased root biomass and increased levels of biological activity in soil.
The majority of Australian soils have lost enormous quantities of organic carbon and this process needs to be reversed. What has gone up must come down. Soils, plants, animals and people will benefit when we take ‘recycle and re-use’ to the next logical step and recycle the excess carbon currently in the atmosphere.
Carbon and nitrogen
Nitrogen moves between the atmosphere and the topsoil in similar ways to carbon. The main difference is that the ‘way in’ for atmospheric carbon is via green plants whereas the ‘way in’ for atmospheric nitrogen is soil microbes. Soils acting as net sinks for carbon are usually also acting as net sinks for nitrogen. The flip side is that soils losing carbon are usually losing nitrogen too. In poorly aerated soils, some of this loss is in the form of nitrous oxide (N2O), a greenhouse gas up to 300 times more potent than carbon dioxide, while other losses include easily leached nitrate (NO3-) which often takes calcium, magnesium and potassium with it, leaving the soil more acidic (lower pH).
Rewarding landholders for farming in ways that build new topsoil and raise levels of soil carbon and nitrogen would have a significant impact on the vitality and productivity of Australia’s rural industries, reduce the incidence of dryland salinity and soil acidity – and reduce levels of greenhouse gases.
As a bonus, regenerative farming practices result in the production of food much higher in vitamin and mineral content and lower in herbicide and pesticide residues than conventionally produced foods.
A new era
If landholders were rewarded for regenerative practices that aggregate rather than aggravate soil structure, it would move us a long way towards solving the greenhouse gas ‘problem’, without the need to measure soil carbon levels at all. Any farming practice that improves soil structure is building soil carbon. When soils become light, soft and springy, easier to dig or till and less prone to erosion, waterlogging or dryland salinity - then organic carbon levels are increasing. If soils are becoming more compact, eroded or saline – organic carbon levels are falling.
Water, energy, life, nutrients and profit will increase on-farm as soil organic carbon levels rise. The alternative is evaporation of water, energy, life, nutrients and profit if carbon is mismanaged and goes into the air.
It’s about turning carbon loss into carbon gain.
Getting started in lifeless, compacted soils where the soil engine has shut down is the hard part. The longer we delay, the more difficult it will be to re-sequester soil carbon and balance the greenhouse equation
Our soils were rich in carbon, life’s treasures flourished there
Then soil health we neglected: earth’s carbon moved to air
Everywhere climates are changing
The future’s becoming unclear
Warming us slowly with carbon
There is no time now to bargain
………………………………………………………………………….
Further information:
‘Managing the Carbon Cycle’ Forums will be held in Horsham, VIC, 26-27 July 2006; Katanning, WA, 2-3 August 2006 and Kingaroy, QLD, 25-26 October 2006. See www.amazingcarbon.com or contact Christine@amazingcarbon.com